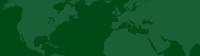
The global population has faced steady growth at around 1% annually, which translates to around 67 million new people every year. At this rate, in 30 years the world’s population will increase by 2 billion people — yielding a total of 9.7 billion people by 2050.[1] Many of these people rely entirely on the agricultural industry to provide a steady supply of sustenance. However, the task of feeding a growing population is daunting, as the available land space for agriculture is limited. As a result, the massive agricultural complex relies heavily on using the available space and food supply as efficiently as possible; this requires minimizing waste at every point in the supply chain, from water usage for irrigation to the hundreds of billions of pounds of uneaten food found just within American landfills alone.[2]
Agritech, or agricultural technology, is an important part of increasing the efficiency of any agricultural process. Earlier, the Industrial Revolution contributed to this by replacing manual labor with machinery. Today’s revolution in connectivity and computing offers agriculture another layer of operational efficiency — for instance, soil moisture sensors to track when to water crops, minimizing any wasted water. There are many use cases where traditional agricultural tasks can be augmented with modern technology to increase yield and minimize waste.
All of these technologies, however, must be properly ruggedized in order to function within these environments ― including their connectors. Connectors within these environments will often be subjected to the outdoors and will therefore experience extensive UV radiation and exposure to the elements such as rain, wind, snow, etc. This means that the connectors used within these applications must have a wide operating temperature range, be composed of materials that will not break down rapidly in the sunlight, and, possibly most importantly, will not fail prematurely due to moisture or dust ingress. This article describes some of the challenges within the agricultural industry today, how Agritech helps overcome some of these hurdles, and, finally, how electronics within these applications ― particularly connectors ― must be ruggedized in order to function within these harsh environments.
Challenges faced today within the agricultural industry
In order to keep up with the growing global population, the agricultural industry must produce more food than it ever has before. The issue is compounded with the limited land space ― only 29% of the earth’s surface is land where 71% of that is habitable, of which 48% has been used for agriculture. Humans have already converted ⅓ of the world’s forests to grow crops and raise livestock, causing a dramatic decline in global biodiversity. By the new millennia, this continuous land clearing and increase in global cropland reached a peak and is now finally beginning to decline and plateau (Figure 1).[3] However, the ever-present demand for food has not decreased — calling for innovative agricultural solutions that optimize the use of existing resources while also minimizing food wastage.
Figure 1: Global agricultural land use has peaked while global food production continues to increase. Source: OurWorldinData.org
Agritech and innovation within agriculture
IoT
Farming and livestock operations have found methods to improve yield with both AI- and IoT-based technologies. The concept of IoT, or the idea of adding sensors to objects, was first discussed in the early 1980s; the term “Internet of Things” was first coined in 1999, although IoT did not gain popularity until 2010. This also coincides with a time where broadband wireless networks were well-established and discussions of next-generation cellular networks (5G) were beginning to form. The first truly discussed applications of IoT referenced inventory tracking and asset management with RFID. However, as everyday consumer devices (e.g., Ring camera, Nest thermostat) become wirelessly enabled for remote tracking and control, the term IoT became more prominent. Wireless sensor networks (WSNs) found their way into smart cities, smart buildings, and even industrial applications with Industrial IoT (IIoT). As the application space grew, so did its respective IoT protocols — some of the most popular of which being those using unlicensed ISM bands such as Bluetooth, ZigBee, Thread, Wi-Fi, and those using licensed spectrum such as cellular connectivity (e.g, 4G LTE, 5G, NB-IoT, LTE Cat M1) for Agritech. Another more recent development was the use of low-power, wide-area networks (LPWANs) that use ultra-narrowband (UNB) technology in the sub-1GHz spectrum to increase the link budget afforded to the system. This allows for small packets of information to be sent over vast distances up to 15 kilometers ― a function that is eminently useful for the large swaths of land used for livestock and crops. A comparison of various LPWANs can be viewed in Table 1. Geolocation systems with GPS and GNSS are also crucial in Agritech for precise field mapping ― and equipment tracking to optimize agricultural operations.
Table 1: A comparison of different LPWAN technologies.
The combination of all of these IoT protocols allows farmers, cattle workers, and other agriculture-based workers to more easily perform their respective tasks. Some potential parameters that can be monitored are seen in Figure 2 where pressure, gas, temperature, and humidity sensors and location tracking via Bluetooth beacons or GPS can be accomplished.
Figure 2: Some of the parameters that can be monitored in smart agricultural applications.[4]
Systems that monitor soil conditions such as moisture, salinity, pH, temperature, and leaf wetness can be easily placed at predetermined locations to relay parameters to a gateway and the cloud for more data analytics. Using longer-ranged wireless networks also releases farmers from the restraints of other IoT protocols. After processing this information, irrigation controllers can then either override scheduled irrigations or perform a previously unscheduled watering of the crops. This is a potential breakthrough technology for irrigation since 70% of all freshwater withdrawals globally are for agriculture.[5] So, agriculture is facing not only a land scarcity but also a potential water scarcity. The efficient use of freshwater is therefore a high priority. Other examples for agriculture-based IoT systems can be found with livestock with collar tags to deliver information such as temperature, heart rate, and blood pressure to gain insights into cattle fertility, digestion, and health via data processing. Sick animals can then, for instance, be physically separated from the herd. Image-based systems such as drones for monitoring (e.g., weed differentiation) and spraying crops might require a larger bandwidth protocol in order to analyze the images being captured in real time.
Sensor systems are central to the functionality of agritech and enable much more efficient farming systems. These systems come with their own engineering considerations and where connectors ranging from wire-to-wire, board-to-board, and wire-to-board may require ruggedization from moisture, dust, and corrosive chemicals. To learn more on connector constraints for sensor systems, read “Engineering Connector Considerations for Sensor Applications.”
Machine learning
The use of machine learning (ML) is closely tied to the use of IoT within agriculture. The massive installation of sensor nodes and parameters yields very useful and novel insights into farming operations where data analysis can be performed for pest, soil, livestock, crop, water, management, and disease and weed detection, as well as yield predictions.
Diseases in crops can be caused by various types of pests, bacteria, fungi, as well as viruses, and can typically be detected by the presence of pathogens or be visually observed (e.g., leaf/fruit spots, color change, wilting). ML algorithms can be used for the early detection of disease in various plants. One paper, for example, uses a proposed dilated and inception convolutional neural network (DICNN) as well as activation-reconstruction generative adversarial networks (AR-GAN) to identify cucumber leaf diseases with image captures (Figure 3).[6]
Figure 3: The proposed approach for identifying cucumber leaf diseases. By obtaining lesion images to identify the precise disease afflicting the plant. Source: [6]
A weed detection and management application can use unmanned ground and aerial vehicles (UGVs and UAVs) to detect and differentiate between the various weeds in a crop and is able to create a map with the location of said weeds. This allows for the precise application of herbicides in specific areas as opposed to spraying entire fields long-term, an expensive endeavor that eventually causes weeds to become resistant to the herbicide.[7]
Challenges for electronics and connectors in agriculture applications
All of these systems will be subjected to the elements and must therefore endure moisture and dust ingress and UV exposure. Other potential stressors include damage from mechanical strain, such as vibration and shock, which might occur within a UAV or heavy machinery used for farming, such as tractors, excavators, combine harvesters, and so on. Electrical connectors within agriculture applications vary broadly from the PCB connections used within smaller sensor modules and drones to the interconnects used with much larger systems, such as heavy equipment.
Contaminant ingress failure mechanisms and solutions
However, all of these use cases will have connectors that must deal with highly damp, humid environments with plenty of dirt. One of the main considerations for connectors in moist environments is the potential that the insulating materials used within the connector housing might absorb the water/water vapor and begin to leak current between the conductors due to the introduction of contaminants. This can also lead to a phenomenon known as hygroscopic swelling, where polymers such as epoxies, thermoplastics, and silicones will expand and exhibit changes in their material properties (e.g., change in elasticity). This will degrade the performance of the connector head, causing unwanted attenuation. In some cases, electrolytic corrosion will begin to occur; this causes a spike in the resistance of the contacts and ultimately failure. In general, any and all contaminants should be kept from the mating surfaces of the electrical connectors. The metal base materials of the pins and housing will more easily react to any corrosive agents. In other cases, dust ingress will prevent the surfaces of the pins from mating properly and degrade connector performance. Agritech may also need to consider the intrusion of chemicals such as pesticides and herbicides and ensure any sensitive electronics/connectors are adequately protected.
Solutions to this issue include using proper gasketing and sealants around the entire connector to prevent moisture ingress. The ingress protection (IP) rating system is often used to classify the level of protection an electronic device has from both dust and moisture ingress. An image illustrating these classifications can be seen in Figure 4.
Figure 4: A guide for IP ratings. Source: IEC
The connector pins can also be ruggedized against contaminants that may cause corrosion (e.g., saltwater). Typically, corrosion-resistant metals such as gold are used to plate connector pins. This is great for electrical continuity and for a mate with a low contact resistance. However, gold is a porous metal and can therefore allow contaminants to slip through the cracks and react with the materials beneath the plating surface. Thicker plating densities can help mitigate these issues and improve the corrosion resistance of the mate.
Mechanical strain failure mechanisms and solutions
Failure due to mechanical strains can also occur; this is especially true within vehicles where the internal circuits/connections will be exposed to constant vibrations. Firstly, the connector head must have a high retention strength. Many connectors have mates that have no way to secure the connection, such as a latch, bayonet-style mate, screw locks, or a threaded mate. This is crucial for a high-vibration environment, as the connector will not hold in place and may disconnect. The other failure mechanism occurs at the connector pins; these mated pins will rub against one another frequently and begin to rub off the material from each of the pins’ surfaces. This is known as vibrational fretting. Once enough material has been rubbed off, the problem will only accelerate as the freshly exposed surfaces will now be much rougher and more susceptible to corrosion.
A thicker gold plating (30µ” or more) can help with this type of fretting and extend the lifetime of the connection under vibrational strain. A contact design that allows for a bit of “float,” or movement, when mated will also ensure there is less rubbing between the metal surfaces of the pins. Connector heads that have a high retention strength will have some kind of mating mechanism that holds the mate in place. Therefore, disconnects will not occur unintentionally and cause system failure.
Inline connectors for in-vehicle connections
As stated earlier, the electronic systems within heavy-duty equipment will be exposed to dirt, water, and chemicals such as hydraulic fluid and engine oil. Vibrations will also occur from off-roading from a dirt path, gravel road, or any rough terrain. Mechanical shock, and possible crushing, can occur from an accident. Both of these types of mechanical strain can cause a slow degradation or immediate damage and failure. Heavy-duty equipment will leverage wire harnesses to connect the many signals required for the engine control unit (ECU) to process and communicate using the CAN protocol. As shown in Figure 5, the wire harness will connect to a number of sensors and actuators through the vehicle as well as the power supply. Typically, the ECU is mounted on (or near) the diesel engine and will therefore be exposed to high temperatures and aggressive vibrations, requiring the wire harnesses to necessarily be resistant to these factors.
Figure 5: Diagram of the piping and wiring system within a dual-fuel supply for heavy-duty equipment. Source: [8]
Inline connectors are optimized for wire harnesses and have a high retention strength as well as a high resistance to vibration. The 560 series inline connectors with double-latch technology were tested for vibration according to MIL-STD-202G 201; during the test, the sample did not fall off, rupture, or damage, and the connector joint did not separate (Figure 6). A retention-force test was also performed by pulling the connection apart at a rate of 25 mm/min with a tensile-testing machine, resulting in a retention force of around 10 kilogram-force (kgf).
Figure 6: The 560 series inline connectors were tested for vibration according to MIL-STD-202G 201 and attached to three axes for two hours on each axis at 10Hz-55Hz-10Hz with a maximum amplitude of 1.52mm.
This resistance to vibrations is due to the connectors. Plastic latches are seated on the top and bottom of the receptacle connector and slid into the housing until they reach the slots for a solid hold. Through EDAC’s E-seal technology, inline connectors feature an IP67 rating, making these connectors completely dust-tight and protected against temporary immersions in water (Figure 7). The connectors also have an extended operating temperature from -40° C to +105° C to help endure thermal shock from the diesel engine.
Figure 7: The inline connectors feature a double-latch technology as well as an IP67 rating.
Card edge, header, and D-subminiature connectors for agricultural IoT applications
The use of sensor modules to monitor various agriculture processes is now entrenched in the daily operations of farms. These modules will need to be sized and ruggedized according to their environments. Wearables used for monitoring livestock will have less space flexibility than a soil condition sensor. However, sensors for soil monitoring will naturally require enclosures and connectors that will not be impacted by both moisture and dust. These embedded systems will generally require board-to-board and wire-to-board connections to realize a multi-PCB solution. EDAC offers ruggedized card edge, header, and D-subminiature (D-sub) connectors that might be used in these applications.
Card edge
Card edge connectors are used to connect the edge of a PCB with a specific exposed copper pattern, or a PCB “electrode,” to a board. This type of connector is often used to add on capabilities to an existing unit. Sensor modules might include card edge connectors to add various types of sensing and protocol capabilities to the existing unit. With over 350,000 standard styles and custom variations of card edge connectors, EDAC is an industry leader in these types of connectors. Customers can select a card edge connector based on the number of contact spacings, type of termination, plating thickness, and mounting style. For vibration-resistant connections, the E-met metal-to-metal card edge connectors are ideal. However, EDAC’s high-temperature card edge connectors will be able to withstand the thermal shock that comes with UV exposure.
The E-met family incorporates EDAC’s hermaphroditic contacts that offer a current rating of up to 10 A per contact. As shown in Figure 8, these contacts use a fork-like shape to achieve a gas-tight mate that floats in the insulator. This allows for micro-movements that mitigate the risk of a vibration-fretting failure.
Figure 8: EDAC’s E-met metal-to-metal card edge connectors diverge from the traditional bellow-style contacts with fork-like pins that achieve a gas-tight mate that holds despite vibrations.
The high-temperature card edge connectors use high-temperature insulators such as Diallyl Phthalate or Polyphenylene Sulfide to perform over an extended temperature range from -40o C to 125o C. This is critical in high-temperature environments where direct sunlight to an enclosure might cause connector failure.
Header
EDAC offers standard box header connectors as well as a ruggedized variant known as the E-pin male headers and E-socket receptacles. These connectors offer a minimum of 200 mating cycles where the receptacle pins are characterized by a fork-like shape that can grab header pins with a high retention strength (Figure 9). This allows for a resistance to vibration as well as a high number of mating cycles. These connectors are also available with high-temperature materials for additional ruggedization.
Figure 9: EDAC’s E-pin and E-socket connectors offer over 200 mating cycles and a high resistance to vibration.
Waterproof D-sub
D-sub connectors are often used for serial communications (e.g., RS-232, RS-485), parallel-port communications, as well as display connections (VGA). These are often used in sensor systems for processing sensor data and communicating it to a processing unit. EDAC offers a wide array of D-sub connectors including standard, machine, high power, parallel port, and waterproof variants. The waterproof D-sub connectors (D-Seal) can withstand immersions and are completely sealed against any dust ingress with an IP67 rating. These connections must be resistant to both dust and water ingress, as these connections are often made on the outside of the sensor module’s enclosure or to connect to a display or human-machine interface (HMI) (Figure 10).
Figure 10: The GT25 rugged HMI from Mitsubishi Electric with RS-485 (3) and RS-232 (4) D-sub interfaces. Source: Mitsubishi Electric
As shown in Figure 11, these connectors are completely epoxy-sealed by potting the leads in a mold and contain a silicone O-ring to meet the waterproof rating. This type of seal limits the risk of epoxy wicking up leads and causing continuity issues.
Figure 11: The waterproof D-sub connectors have the entire back of the connector sealed rather than the individual pins to yield a complete seal with a smooth finish.
Waterproof HDMI and USB connectors
HMIs in rugged agricultural applications will have to consider rough handling in a farming-based work environment — an environment where the user may not have clean hands or a steady, gentle grip when handling a touch panel — or smart flight controllers for agriculture drones. D-sub and HDMI connectors are the connectors of choice for HMIs. USB connectors are prolific and often used to send or receive data from a handheld device such as portable test equipment or a laptop ().
EDAC offers waterproof HDMI and USB connectors with an IP67 rating (Figure 12). Optional dust caps can keep contaminants out until the connector is mated in the field. These connectors feature a circular housing with a secure quarter-turn twist and lock-latching system for a high retention strength that is vibration- and shock-resistant. This diverges from traditional HDMI and USB solutions that have no latching mechanisms and will likely disconnect or become dislodged in the event of mechanical strain.
Figure 12: EDAC’s waterproof, IP67-rated connectors come in USB type A, micro, and mini-B, as well as HDMI.
Conclusion
EDAC offers a massive range of connectors that are suitable for many types of agritech. These connectors are able to meet the demands of the agricultural environment with ruggedization that prevents damage from vibration, mechanical shock, as well as damage from contaminants such as water, debris, and chemicals. Many of these connectors are also available with high-temperature materials in order to withstand the thermal shock that the electronics may endure when exposed to direct sunlight for prolonged periods of time. From heavy-duty equipment to IoT, EDAC has the legacy and expertise to provide the optimal connector for the application.
References
- United Nations Department of Economic and Social Affairs. “Global Issues: Population”, https://www.un.org/en/global-issues/population.
- Feeding America. "Food Waste in America." Feeding America, 2023, www.feedingamerica.org/our-work/reduce-food-waste.
- Roser, Max, Hannah Ritchie, and Esteban Ortiz-Ospina. "Peak Agricultural Land." Our World in Data, 2022, ourworldindata.org/peak-agriculture-land.
- M. S. Farooq, S. Riaz, A. Abid, K. Abid and M. A. Naeem, "A Survey on the Role of IoT in Agriculture for the Implementation of Smart Farming," in IEEE Access, vol. 7, pp. 156237-156271, 2019, doi: 10.1109/ACCESS.2019.2949703.
- World Bank. "Water in Agriculture." World Bank, 2023, www.worldbank.org/en/topic/water-in-agriculture.
- Zhang J, Rao Y, Man C, Jiang Z, Li S. Identification of cucumber leaf diseases using deep learning and small sample size for agricultural Internet of Things. International Journal of Distributed Sensor Networks. 2021;17(4). doi:10.1177/15501477211007407
- Benos, L.; Tagarakis, A.C.; Dolias, G.; Berruto, R.; Kateris, D.; Bochtis, D. Machine Learning in Agriculture: A Comprehensive Updated Review. Sensors 2021, 21, 3758. https://doi.org/10.3390/s21113758
- Zhou, H.; Zhao, H.-W.; Huang, Y.-P.; Wei, J.-H.; Peng, Y.-H. Effects of Injection Timing on Combustion and Emission Performance of Dual-Fuel Diesel Engine under Low to Medium Load Conditions. Energies 2019, 12, 2349. https://doi.org/10.3390/en12122349